DIGITAL MATTER – INGEO
Ingeo project focuses on the development and optimization of material system based on modified Ion- Exchange Resin (IER). This material has Carbon Dioxide (CO2) absorbing capabilities, and therefore is able to purify air and cool the atmosphere in our cities. This material is an active part of a performative architectural skin, geometrically enhanced through computational design optimization.
WHY CO2?
Carbon dioxide is a naturally occurring trace gas that is a vital part of Earth’s environmental system. It is also a greenhouse gas that absorbs heat. Consequently, as the level of carbon dioxide in our atmosphere increases, a greenhouse effect develops, trapping additional heat and raising earth’s average temperature. Carbon dioxide concentrations are rising as a result of human activity, such as the burning of fossil fuels, agriculture and construction. Plants are capable of pulling CO2 out of the atmosphere through the process of photosynthesis, however CO2 is being released at a much faster rate. (Woods, 2018).
Probably, two of the largest environmental concerns in cities today are carbon emissions and air quality. Urban zones with intense emissions from vehicles, leading to decrease of air quality, affect human health. As a result, cities are now under increased pressure to demonstrate plans to control and reduce air pollution. – The measurement of carbon dioxide levels in a city canyon – J Boyd, D Budinov, I Robinson, J Jack 2016
METHODOLOGY
To design a performative material system that can absorb CO2 from our surrounding environment.
HYPOTHESIS
Computational algorythms can significantly improve the design and optimization process of Ingeo modules geometry and maximise the efficiency of CO2-absorbing material system.
STATE OF THE ART
We aimed our proposal to be not only a simple device or piece of infrastructure, but add an aspect of art to it. We developed a project, that combines performing its’ function and attracting peoples’ attention. It seems like recently, there are more and more examples of performative facades and materials, serving both as a structure and as a notification about ecological concerns.
TESTING MATERIALS
Initially, we considered three main materials, that obtain CO2-absorbing capabilities. We tested biochar, modified titanium dioxide (TiO2) and ion-exchange resin to find the best candidate for active material in performative ingeo modules.
TESTING APPARATUS 1.0
OPTIMAL MATERIAL
Ion-exchange resin geometry, developed by Klaus Lackner. Photo by Celine Grouard
While selecting an optimal material for CO2 absorption, we evaluated each of them according to the following parameters:
- CO2-absorbing capabilities
- Reusability
- Ability to take a needed form / coat the geometry
- Period of degradation – cleaning / processing / manufacturing / duration
- No production of harmful byproducts/chemicals
- Attainability/affordability
- Its processing must not contradict its CO2 absorbing abilities
Based on the listed parameters, ion-exchange resin appeared to be the optimal candidate.
ION-EXCHANGE RESIN
IER is a polystyrene sulfonate and comes in the form of translucent yellow microbeads. The main application of IER is water filtration or purification due to its ability to exchange ions. However, professor Klaus Lacker was the first person to utilize these abilities for air filtration by modifying the IER. The modification process includes dipping the material in a Sodium Hydroxide solution, which gives IER particles the ability to absorb CO2 from the surrounding air. By changing the physical state of the IER beads via process of melding and binding the loose particles with polypropylene, Professor Lacker developed a flexible and feasible sheet product which successfully absorbs CO2 from the ambient air with relatively low CO2 concentration.
POLYMER BINDERS
We conducted several tests to pick an optimal binder material, melting various polymer beads with tetrahydrofuran and mixing the solution with IER particles. First of all, the IER microbeads were ground to a powder using a pestle and mortar. After that we prepared a solution, dissolving polymer in tetrahydrofuran. The following step was mixing IER with the polymer-based solution. Finally, we poured resulting solution in petri dishes to let material solidify and test resulting forms.
According to our experiments, the weakest polymer solutions were the ones containing Styrofoam, ABS, and Z-glass. In contrast, the strongest and the most durable polymer coatings and films were those based on PVC and Polycarbonate.
MATERIAL SYSTEM CYCLE
The cyclic protocol of the IER material system consists of a series of steps. First of all, the base geometry is dipped in a polymer coating, which contains 10% of the IER, 10% of PC and 80% of tetrahydrofuran. According to our experiments, the geometry, when made of the same solution polymer and then coated in the solution, leads to melting of coating and the surface polymer layer. When the coating gets dry, the resin requires a Sodium Hydroxide (NaOH) solution wash, to activate the CO2 absorbing capabilities. After soaking in the NaOH solution for 1 hour, the geometry becomes capable of absorbing Carbon dioxide. The shown color indication of CO2 absorption is highlighted in 7.8 Method of Materializing Data.
The geometry was tested in Apparatus 1, a sealed glass bell with a fan and CO2 monitor. The resin, saturated with CO2 requires incremental Sodium Carbonate (Na2CO3) solution washes to unload, capture, and transport the collected CO2. Most importantly, the system is capable to absorb CO2 thereafter for numerous cycles.
Na2CO3 itself is a water-soluble salt. Simple water (H2O) also unloads the resin, however, in contrast to Na2CO3 solution, does not hold onto the CO2 molecules. As a result, water wash makes the CO2 evaporate back into the atmosphere. Our washing tests are conducted on the geometry in Apparatus 2, a seal glass tank with a water pump cycle, assembled around the geometry.
MATERIALIZING DATA
Bromothymol blue (C27H28Br2O5S) is a pH indicator that changes color in the presence of an acid. The range of BB is between 6 and 7.5 pH. BB will maintain a blue color with levels of pH above 7.5. pH, while levels below 6 will initiate a change to green and continue to change to yellow with pH levels below 5. BB has been used as an indicator for CO2 levels in the water. The CO2 reacts with water (H2O) to form carbonic acid, lowering the pH and therefore changing the color from blue to yellowish green.
As a result, this system of physical color change can perform as an indicator to initialize the washing of a saturated facade. Beginning with a bluish hue, the panel color will change to a green/yellowish hue as it absorbs CO2. Not only is this an indicator to unload a saturated facade, but also a method of materializing data, physically indicating the current surrounding air quality and rate of pollution saturation.
TESTS: COATINGS + FILMS + SOLIDS
We conducted numerous experiments to select the optimal material format. The images below represent the most important results, received during these tests.
Among the films, coatings and solid pieces the coating has shown the best weight-to-performance ratio. As the CO2 molecules are absorbed when in contact with surface, the active material layer can be thinner than a film, but must be applied to a stronger material to make the entire system self-bearing.
LIQUID EXTRUSION TESTS
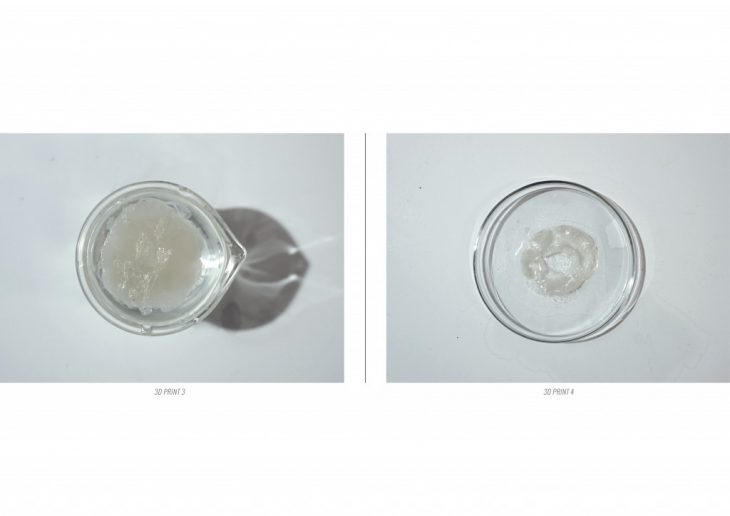
FORM-FINDING GEOMETRIES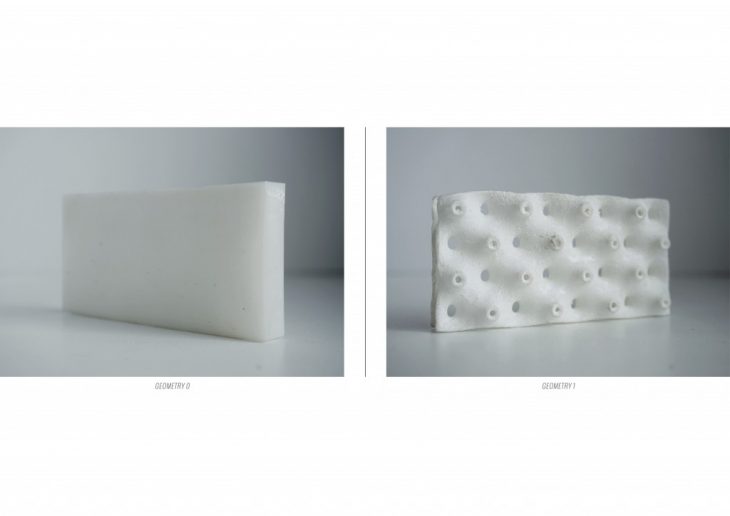
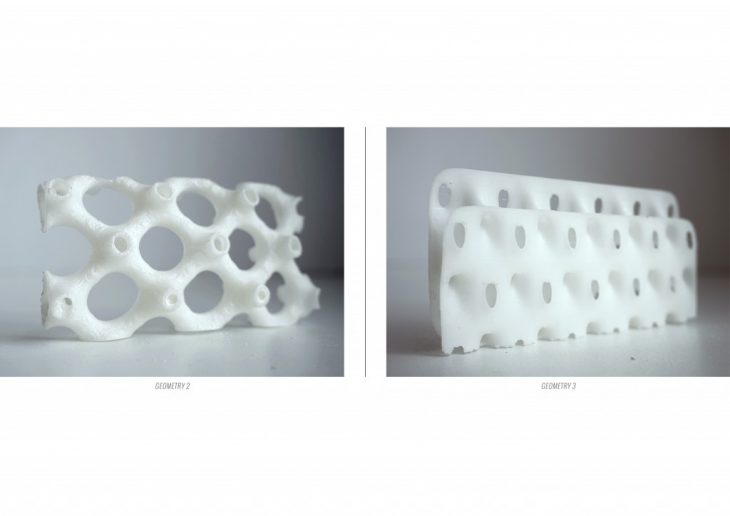
DESIGN DRIVERS
UNLOADING CO2 CYCLE
Once the resin is saturated with CO2, a sprinklers system initiates the incremental washes to unload the CO2 from the panel. We tested the unloading system inside the washing apparatus. A tank full of sodium carbonate solution installed at the top of the panelized facade, releases the solution through the panels, using gravity to wash the vertical waved surfaces of the interior panel walls. The seamless connection from panel to panel, allows the washing solution to continue towards a collection pipe in the bottom panels, which then leads to a basement tank. In order to prevent the “water hammer” effect, the facade is vertically divided into several sections, up to 10 meters high each.
A pipe leads from the bottom of each sections to the basement tank, where CO2-saturated solution is collected and filtered, to use a regenerated solution again. The Na2CO3 solution and the collected CO2 are then able to be removed and processed. The processing takes the sodium carbonate wash solution, plus its absorbed CO2 combined with water and heat and results with an output product of NaHCO3 (Sodium Bicarbonate). Using the reversed process, these NaHCO3 salts could be utilized to create more sodium carbonate solution to begin the cycle again. The processing of extracting Na2CO3 from NaHCO3 would require controlled processing to ensure the captured CO2 does not return back to the atmosphere.
WASHING APPARATUS 2.0
OPTIMIZATION – SIMULATION DESIGN DRIVERS
To increase the performance of the material system and the carbon dioxide absorption rate, it is necessary to create a geometry that fits the following parameters:
1. The maximum active surface area to increase the amount of carbon dioxide absorbed per cycle.
2. The maximum surface area to increase the amount of performative material that can be applied.
3. The maximum contact of the active surface with ambient air to accelerate carbon dioxide absorption per cycle.
4. Protection of the active surface from rainwater to prevent the release of carbon dioxide into the ambient air.
5. Minimum consumption of sodium carbonate solution with evenly washing of the panel per cycle.
MODULE GEOMETRY OPTIMIZATION
MAXIMIZED SURFACE AREA OPTIMIZATION CATALOGUE
INTEGRATED WASHING INFRASTRUCTURE CATALOGUE
MAXIMIZED SURFACE AREA OPTIMIZATION INTEGRATED WASHING INFRASTRUCTURE CATALOGUE
MODULE IN LAYERS
We propose to manufacture Ingeo modules through the process of vacuum forming Polycarbonate sheets, on which we apply our performative material coating. 3D-Printing complex geometry is also an option to apply the material coating, however, this method would have a much higher manufacturing cost. By designing the geometrically optimized surface of our module sheets, we are able to develop the form and digitally fabricate the molds through CNC milling. Three molds are made with allocated geometry for the front, middle, and back sheets. After manufacturing, the three vacuum-formed polycarbonate sheets will join together to create 1 Ingeo module.
ARCHITECTURAL PROPOSAL
SECOND SKIN / FACADE
The modular aspect of Ingeo allows it to be adaptive and installed on blank facades of existing buildings, most preferably those that exist in highly-contaminated areas (e.g., parking, city towers, trade centers). This process requires not only the installation of panels but also the creation of an additional washing and CO2-separation system inside the basement of the building. This washing system is referenced in context 8.0 Unloading CO2 Cycle.
This application of Ingeo brings utility and performance to unused infrastructure. The application helps to purify the air inside and around the building, with extra thermal insulation and cooling abilities to the facade, which is especially useful in hot and dry regions. Applying Ingeo panels can significantly improve the ecological certification rating (LEED, BREEAM). This solution not only provides an initiative for a more sustainable and green city. Furthermore, it has a potential economic benefit in reducing the carbon tax of a building.
INTERIOR PARTITION / WALL
The external average level of CO2 is at 380-400 ppm, the average level of CO2 in an enclosed space accumulates and should “be under 1000 ppm, which is equivalent to a concentration of 0.1%.”(Derek J Clements-Croome 2008) However, depending on the ventilation system within the building “carbon dioxide levels [inside] can vary from 800 ppm to almost 5000 ppm.” (Derek J Clements-Croome 2008) Carbon dioxide, in concentrations of 7.5% can lead to headaches, dizziness, restlessness.” (Derek J Clements-Croome 2008) We propose that by including Ingeo modules in office spaces as partitions, we can drastically improve air conditions, health, and productivity within a work environment. According to Derek J Clements-Croome, “Indoor air quality is a vital factor for the health of occupants in buildings. The air we breathe ultimately affects the efficiency and effectiveness of our thinking.”
AIR PURIFYING TOWER
By designing an optimized structure with the integration of Ingeo’s key parameters, we are able to maximize the performance of the shape and structure itself, but also attract public attention to the CO2 issue. The structure consists of 7 high and curved “chimneys”, merged together and covered by Ingeo modules from the inner side. Each chimney is wide at the bottom and becomes more narrow when reaching the top. Such configuration results not only in the maximal surface area but also provides stronger airflow on the inside of the tower, increasing the efficiency of CO2 absorption.
The inner surface is tessellated for further area maximization and better washability of Ingeo modules. The entire volume of Air-Purifying tower is optimized to minimize the shade created by the building and its’ influence on the city silhouette. In order to attract people’s attention to the air contamination and to visualize the data of CO2 levels, the Ingeo modules of the tower are coated in a special solution, which changes color depending on the CO2 levels in the air.
DATA ANALYSIS
GENERAL INFORMATION
A single person exhales 0.8 – 1.1 kg of CO2 per day.
1 mole (44 grams) of CO2 are produced for every 400 kJ of energy produced (on average).
Average concentration of CO2 is 400 ppm, and can rise up to 3000 ppm in closed spaces.
At city streets with intense traffic CO2 concentration is up to 1000 ppm.
INGEO PANELS’ PARAMETERS
1 Ingeo panel absorbs approx. 200 grams (0.2 kg) of CO2 per day.
Panel gets saturated with CO2 for 1-5 hours (depending on airflow).
Surface area of the panel is 1.98 m^2, while it takes only 0.5 m^2 of facade surface area.
During 1 year a single panel can absorb up to 140 kg of CO2 per year.
SYSTEM ECOLOGICAL FOOTPRINT – CONTENT
- Tetrahydrofuran – derives from a byproduct of dehydration agricultural waste and biofuel.
- Plastic particles – can come from any source, from bioplastic to recycled ABS plastics.
- Ion-exchange resin – derives from polystyrene.
- Sodium carbonate – produced from limestone and sodium chloride.
- Sodium hydroxide – produced from sodium metal and water.
SYSTEM ECOLOGICAL FOOTPRINT – ENERGY CONSUMPTION
- 1 mole (44 grams) of carbon dioxide is produced to create 190-1050 kJ of energy (depending on the method of energy generation).
- Approx. 100 kj is needed to maintain one panel performance – 0.1 – 0.25 moles of carbon dioxide is produced in the process.
- Consequently, the system maintenance emits up to 20 times less carbon dioxide than it absorbs.
INGEO is a project of IaaC, Institute for Advanced Architecture of Catalonia developed at Master in Advanced Architecture, in 2018/2019 – * PATENT PENDING*
Students: Olivia Alvarez, Anton Koshelev, Ivan Marchuk
Faculty: Areti Markopoulou, David Leon, Raimund Krenmueller
Material expert: Giovanni Perotto
Student Assistant: Nikol Kirova
Special thanks to Prof. Klaus Lackner and his colleague Allen Wright, for all their help and providing us with information and material samples for experiments.